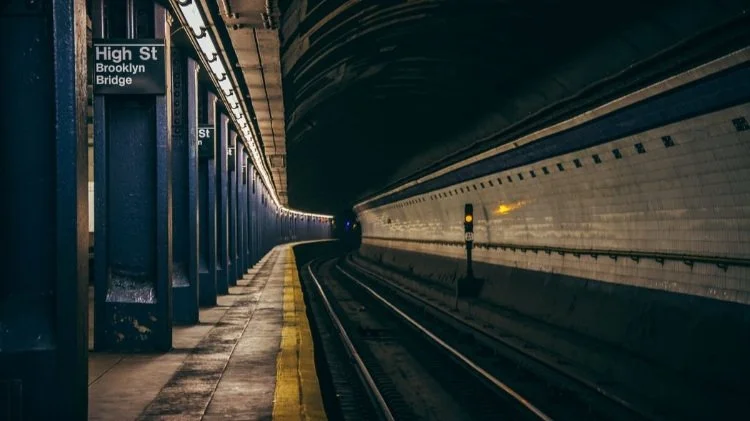
OUR RESEARCH
Stroke is the leading neurological cause of disability worldwide. Stroke frequently devastates movement, limiting the ability to care for oneself. Despite our best therapeutic efforts, recovery is generally incomplete and insufficient. We believe that our therapies can and should do better.
Our mission is to restore motor function after stroke by harnessing the brain's neuroplastic potential. We focus on treatments to accelerate motor recovery and on the mechanisms that underlie this acceleration. Our research seeks to hone neurorehabilitation strategies, guided by a deeper understanding of neuroplasticity and motor learning.
mechanisms of neurorecovery
How does the brain re-learn to move skillfully after stroke? A major area of research in the lab is understanding how neural pathways support motor recovery. We use noninvasive brain stimulation (e.g. transcranial magnetic stimulation) and neuroimaging (e.g. diffusion and functional imaging) to examine the functional and anatomical connectivity of these circuits after stroke. This multi-modal approach not only enables us to identify the major pathways that drive motor recovery, but it also helps us build rational therapies to assist these pathways and drive recovery.
measurement of rehabilitation dose & recovery
Another major area of research is developing new tools to objectively measure training dose and movement quality in individuals undergoing stroke rehabilitation. Animal models have long shown that high training doses after stroke produce greater motor recovery. In humans with stroke, the optimal rehabilitation doses are unknown. This is because we have no easy way to count arm movements and their quality during rehabilitation, so we don’t know how much and how well individuals are doing. To solve this measurement problem, we use artificial intelligence to automatically identify and assess movements from their patterns in sensor- and video-based motion data. These measurement tools will be critical for rigorous dose-response rehabilitation trials in the future.
brain stimulation for neurorecovery
A third area of research in the lab is using brain stimulation to boost plasticity in brain and spinal networks after stroke. Brain stimulation can encourage or reinforce the plasticity elicited by physical training, resulting in larger motor gains. We use approaches like vagus nerve stimulation (VNS) and transcranial direct current stimulation paired with physical training to accelerate motor recovery. Importantly, we interrogate their functional and anatomical effects with TMS and neuroimaging, so that we can identify what works for iterative refinement.